More and more people with unusual diseases are diagnosed
In just a few years time, researchers have obtained tools to diagnose a large number of diseases that were previously unknown. Now the hope is that this diagnostic revolution will also lead to new treatments.
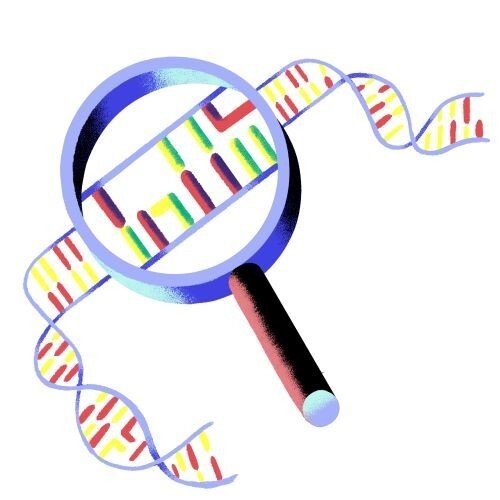
Text: Fredrik Hedlund, first published in the magazine Medicinsk Vetenskap nr 1/2019. Illustration: Amanda Berglund
A rare disease is a condition that affects less than five out of 10,000 persons, or one in 2,000 persons. As a comparison, a common disease such as diabetes affects one in 20 Swedes.
However, there are numerous rare diseases, estimated at between 6,000 and 8,000, which means that in total there are about as many patients with rare diseases as there are diabetics.
“When taken together, rare diseases are very common. It is estimated that between five and seven per cent of the population is affected. This means around 500,000 Swedes,” says Ann Nordgren, Professor of Clinical Genetics at Karolinska Institutet and senior consultant at Karolinska University Hospital.
She is one of two research group leaders for the Rare Diseases research group at Karolinska Institutet and is responsible for Karolinska University Hospital’s “Undiagnosed disease program”. Here, patients with rare syndromes are examined to find out what diagnosis they have.
But even though rare diseases are common when viewed together, they are individually very uncommon and therefore difficult to diagnose. In addition, the tools for distinguishing and identifying the rare diseases, which are often genetic, have not been available. However, there is currently a diagnostic revolution happening in the field of rare diseases, and Sweden is at its forefront. In just a few years, there have been enormous developments within genetic diagnostics. Since 2015, Stockholm County Council has been first in the world to carry out whole-genome sequencing in clinical activities to diagnose patients with ambiguous diseases.
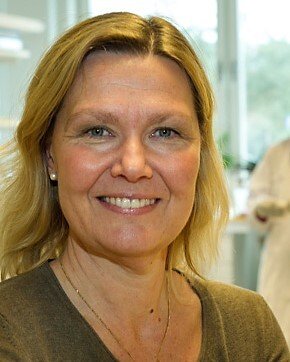
“This is a huge technological leap which means that you can now analyse the entire human genome. Since 2010, we have been conducting intensive development work together with technical experts at the SciLifeLab national centre and clinically active experts at Karolinska University Hospital,” says Anna Wedell, Professor of Medical Genetics at Karolinska Institutet and senior consultant and Director of the Centre for Inherited Metabolic Diseases at Karolinska University Hospital.
She explains what this means for her patients in more concrete terms.
“It can involve everything from suddenly understanding why a long-undiagnosed patient is ill, to identifying a patient on their way to becoming ill, but where we can avoid a severe handicap or save a life by quickly administering the right treatment,” she says.
When treating patients with rare diagnoses, treatment and research go hand in hand. The patients are so rare that each treatment success is a new step forward for research. Often, doctors must also collaborate across county and national borders to find similar patients and doctors with experience of the rare diagnosis.
Wants to give undiagnosed children a diagnosis
Many rare diseases are still undiscovered. The Wilhelm Foundation was founded in 1999 by Helene and Mikk Cederroth, who lost three children to an unknown disease between 1999 and 2002. The children were 16, 10 and 6 years old.
- The aim of the foundation is for all undiagnosed children to be given a diagnosis.
- Since 2014, the foundation has, together with a researcher at the National Institutes of Health, hosted a series of world congresses where experts meet and discuss undiagnosed cases.
- In 2017, the congress was held in Sweden, after which the Karolinska Undiagnosed Diseases Program was formed.
- Nowadays, there is also an international network, Undiagnosed Diseases Network International (UDNI), for experts in undiagnosed diseases.
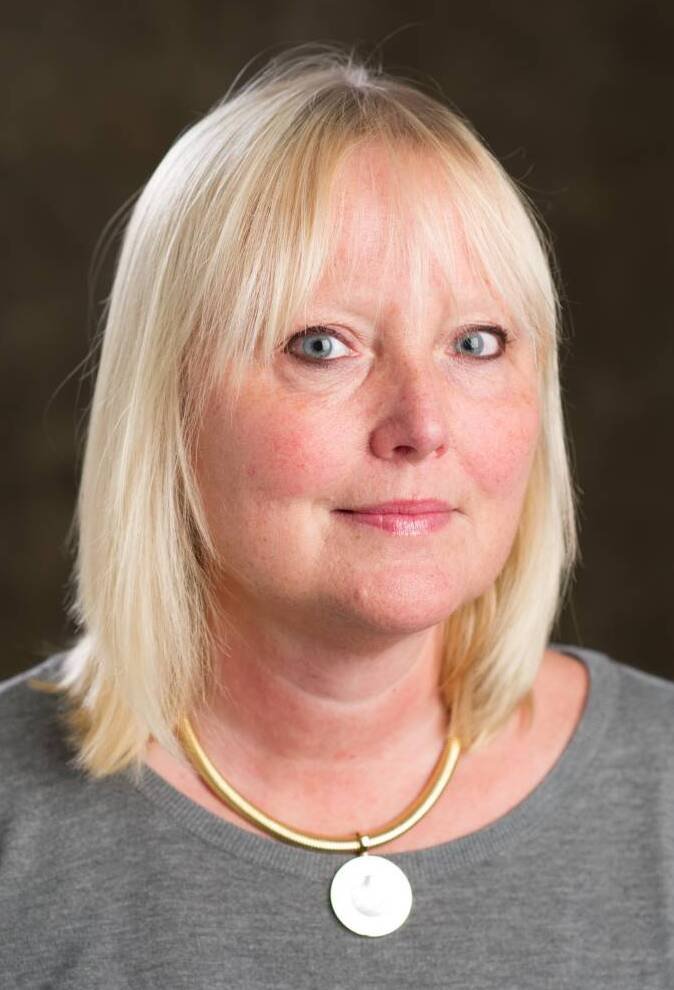
“The Cederroths have done something very important in bringing us researchers together,” says Ann Nordgren.
Ann Nordgren’s specialist field is syndromes, malformations and developmental disorders. She explains that new genetic diagnostic tools have in one swoop improved the level of understanding and insight regarding patients in her field. She takes children with developmental disorders as an example. Children who do not develop normally have previously been lumped together under the umbrella concept of developmental disorder. A few clear and known diagnoses have been established, such as Down’s syndrome, but most have been unclear. However, this has now changed radically.
“Today, there are about 1,000 known genetic causes behind developmental disorders. All are unique and very few are linked to complications during childbirth. The most common cause is a genetic aberration,” says Ann Nordgren.
And the list of genetic causes of rare diagnoses is constantly growing, she says.
“In 1959, we knew of one genetic cause, Down’s syndrome, now the molecular cause is known for well over 5,000 diagnoses. Every week new diagnoses are established,” she says.
The finding of new molecular causes behind rare diagnoses does not mean that they can automatically be treated with drugs. However, understanding the genetic background of a disease is a prerequisite for being able to start work on finding a specific treatment against it, and there is much else that can be done thanks to knowing the cause and gathering knowledge about symptoms and prognoses.
“Treatment need not always be a medicine – it can also be prevention and care,” says Ann Nordgren.
She talks about syndromes with cancer predisposition where a correct diagnosis can lead to tailored surveillance programs and thereby save lives and about patients with Williams syndrome who, among other things, have a lot of anxiety due to their illness. For example, going to the dentist can be experienced as a real nightmare for them. The parents can then go through the process with them in detail before the dentist’s visit so that they feel calmer. In the case of another rare disease, a secondary symptom in some cases is a heart defect. After a diagnosis has been made, the doctors immediately look for the heart defect and can correct it if found.
Many of Ann Nordgren’s patients are young children and it is also very common for the parents to worry about whether they should dare to have more children. Will the genetic mutation also be inherited by the next child? If the genetic background of the disease is known, she can sometimes put the parents' minds at rest with information that there is no increased risk for the next child, or if there is, she can offer prenatal diagnosis for the next pregnancy. With a known mutation, it is possible to offer pre-implantation genetic diagnosis. This involves in vitro fertilisation followed by an analysis of the embryo before being inserted into the uterus, to be sure that the mutation has not been passed on.
A slightly different situation is faced by Anna Wedell, who specialises in inherited metabolic diseases. These diseases are often possible to treat once you find them and understand what is wrong.
“Sometimes it involves dietary treatment, you can add or subtract something, and the treatment can also involve enzymes or drugs,” she says.
Because many patients are treatable, it is particularly important to establish a quick and effective diagnosis.
“It can be an acutely sick child lying in neonatal intensive care where you take a lot of samples and must understand the biochemistry and the neurobiology behind the condition,” says Anna Wedell.
A quick answer is naturally the most important thing for the patient in question, but it also has significance for the research which can progress in its understanding of how the body works.
“Let's say that we have a patient with an undefined illness where their brain is not working as it should, or the child is not developing properly, and then suddenly we find out which gene causes it. This gives us brand new knowledge, we get new puzzle pieces on how the brain works normally and we can start to unravel the biology behind the disease,” she says.
Both Ann Nordgren and Anna Wedell point out that although the new genetic engineering is crucial for the new diagnostics, it is not about sending a sample away and getting a response indicating what is wrong.
“You are presented a long list of genes, and understanding which of all of these causes the disease is then really difficult. We all have mutations in a lot of our genes without becoming ill from it,” says Ann Nordgren.
This is where their expertis in their respective areas comes in. They must be able to link other characteristics of the clinical picture to the mutated gene that is likely to cause the disease, and they do this in different ways depending on their fields. Where Anna Wedell takes a blood sample and measures substances that are present in too high or too low amounts, Ann Nordgren x-rays, measures and observes her patients.
She examines the patient’s body very carefully. She notes body proportions and observe whether the patient has any characteristic facial features.
"This is called gestalt", she says.
What may sound like something left over from a bygone era is in fact a completely necessary analysis, emphasises Ann Nordgren.
“If we don’t do this, we’ll get nowhere with whole genome sequencing. We can often suspect that it should be this or that signalling pathway that’s affected simply by observing the person’s appearance. But in that signalling pathway there can be a lot of different genes. This means that we still have to perform whole genome sequencing to find out which one of all these genes is involved,” she says.
However, the opposite can also be true.
“Many times we have compiled symptoms in this way and still don’t understand what it is. We then send samples for whole genome sequencing and find out that it may be a certain gene, then we go in and read about it, find out about how others with this diagnosis look and what symptoms they have had. The puzzle is pieced together from both directions, from the patient’s symptoms and characteristics and from genetic data,” she says.
And piecing together this puzzle requires a lot of information on different patients, which is of course difficult when patients are rare. Researchers therefore collaborate in networks across the world where they can more easily identify the diseases by sharing data.
“We do this for the patients or parents who really want us to do so. These families are very anxious to find out why they have a child who is ill. We talk through things carefully, after which they sign a consent form if they want,” says Ann Nordgren.
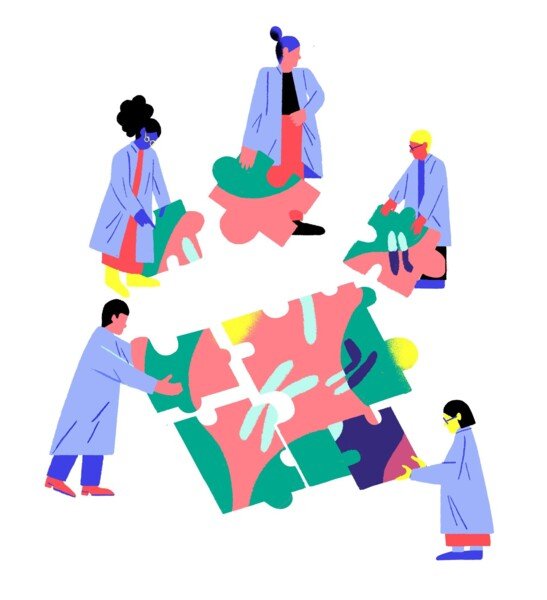
Where Ann Nordgren and Anna Wedell are looking for mutations in individual genes, researcher Anna Lindstrand is looking for changes in entire chromosomes. She is a specialist in clinical genetics and an associate Professor at Karolinska Institutet. She is also the second research leader for the Rare Diseases research group together with Ann Nordgren.
All our genes are divided into 46 chromosomes, which can also have changes that cause diseases. The most well-known chromosomal change is Down’s syndrome, which is due to an extra chromosome 21. This is a genetic defect that can be seen with the naked eye under a microscope.
What Anna Lindstrand is now looking for is, for example, when a segment from one chromosome has switched places with another segment from another chromosome, so-called translocation. Or when a DNA molecule has broken off in two places and the bit between has turned 180 degrees, a so-called inversion. This sounds dramatic, but it does not have to mean anything.
“The most important thing is the location of these breaks. If it is inside or near an important gene, it can lead to disease, but if the breaks are between genes, you can have such deviations without knowing it,” says Anna Lindstrand.
She explains that large parts of the DNA molecule do not contain genes that code for important proteins or the like, so the chance of the breaks ending up between genes is quite high.
But if the breaks end up in a gene, it can actually lead to any type of disease, depending on which gene is involved. Anna Lindstrand’s research therefore does not focus on a particular type of disease.
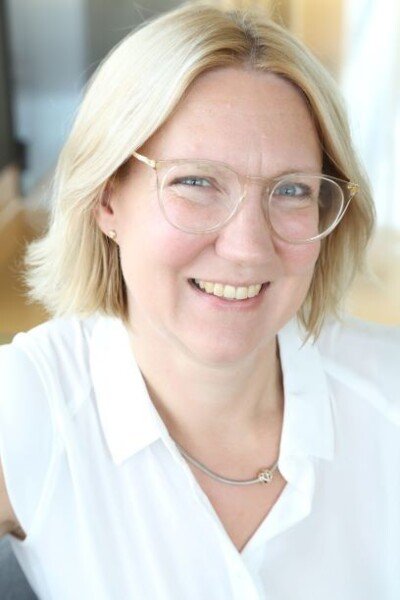
“I’ve always been interested in diseases that affect the brain. In the case of major chromosomal changes, there will often be an intellectual disability,” says Anna Lindstrand.
Instead, she has another speciality; the possibility of evaluating genetic findings in a zebrafish model. It involves determining whether a mutated gene really is causing a given disease. She can do this by knocking out the corresponding gene in the fertilised fish eggs and instead injecting the normal human gene into certain eggs and the mutated gene into others, so that she can follow how the fish embryos develop.
The model is based on the fact that the disease causes some type of malformation that can be read in the fish embryo.
A female zebrafish lays several hundred eggs at a time. In just one trial I can then compare several hundred individuals and get statistics that show whether it is likely or not that this gene is pathogenic.
“A female zebrafish lays several hundred eggs at a time. In just one trial I can then compare several hundred individuals and get statistics that show whether it is likely or not that this gene is pathogenic,” she says.
The zebrafish is one of the animals whose genes are most extensively mapped, and about 70 per cent of the human genes are also present in the fish. In addition, the fish embryos develop very quickly.
“After only 36 hours, the heart starts beating and the brain develops. After five days, all major organs are in place. And since the whole process takes place outside the mother’s body and the embryos are transparent, I can monitor this in my microscope,” she says.
It is also possible to use the fish model as a first step in finding new drugs for the unusual genetic diseases. By allowing the genetically modified fish to grow to adult size, it is possible to test whether potential drug substances affect them.
“This can help us find mechanisms that are important for rare diseases and substances that can be applied further in a mouse model,” says Anna Lindstrand.
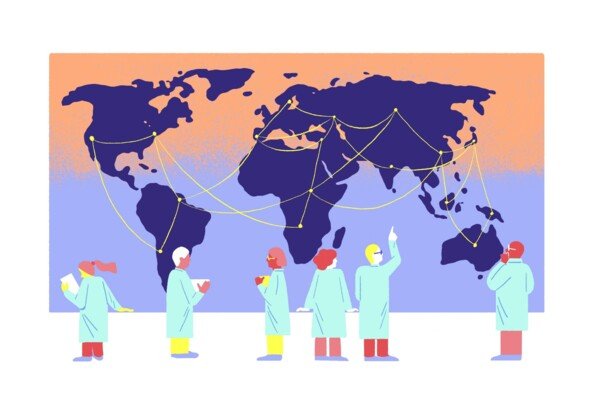
One area Where research has already progressed considerably further is neuromuscular diseases. There are several hundred neuromuscular diseases with a hereditary cause, but most of the interest is now directed towards two of them – Duchenne muscular dystrophy, DMD, and spinal muscular atrophy, SMA. Both are very serious muscular diseases that cause the muscles to atrophy and, in the worst case, lead to death for those affected.
The diseases are due to the mutation of different genes, which means that necessary proteins do not form as they should. Both diseases have recently had new drugs approved by the European Medicines Agency, EMA. The drugs work in slightly different ways, but both intervene and correct the reading of genes so that the necessary proteins are created in sufficient amounts, and in this way can improve the children’s muscle function.
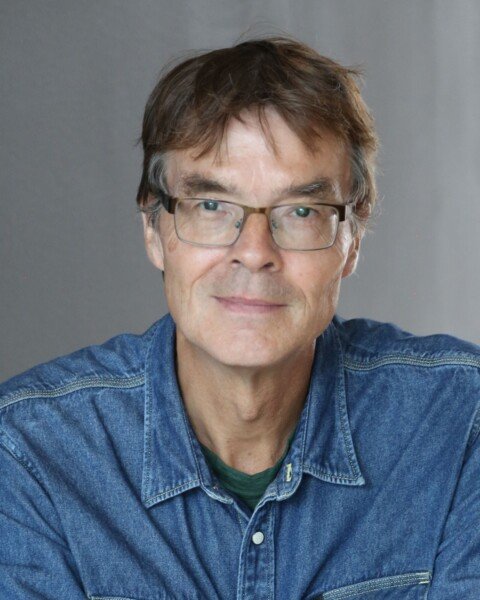
Thomas Sejersen is Professor of Pediatric Neurology at the Department of Women’s and Children’s Health at Karolinska Institutet and senior consultant at Karolinska University Hospital. He has been researching these diseases for decades.
“We had ideas early on that it would be possible to modify the genome in some way, but at times these ideas seemed like mirages to us. But in the last five to ten years, there have been tremendous developments,” he says.
The treatments of Duchenne muscular dystrophy and spinal muscular atrophy can be said to be the first examples of successful therapies for rare diseases that attack the fundamental problem itself, the mutations, and they can be seen as model treatments for the future,” says Thomas Sejersen.
“There is hope that the principles that apply to these can benefit other neuromuscular diseases, and other rare diseases as well,” he says.
The current drug treatments affect the reading of the genes, but they do not alter the DNA molecule itself. In addition, the drugs must be administered repeatedly in order to have a long-term effect. But this may soon be history, suggests Thomas Sejersen.
“Gene therapy has been talked about for decades, but time and time again it has been met with opposition. But now there has been success in modifying the carriers of the genes that we want to introduce, so it looks extremely promising for these muscular diseases. In the US, a small group of children with spinal muscular atrophy have now been successfully treated, and more studies are under way. Approval is expected in the US very soon, and in Europe a little later,” he says
This would mean a one-time treatment that introduces a new functioning gene permanently into the DNA molecule.
Regardless of whether it is a drug or gene therapy, it will be important to identify the children as early as possible before the muscles begin to atrophy. In yet unpublished studies, it has been shown that early treatment can render the children almost symptom-free,” says Thomas Sejersen. He is therefore trying to institute a screening of all Swedish children in the so-called PKU test that is done on all newborns.
“It would be very beneficial if this could be offered as a screening test so that treatment could be administered early for optimal effect,” he says.
Investment in technology is required
The national centre for molecular biosciences, SciLifeLab, which was founded in 2010 on the initiative of the Government, has allowed Sweden to be a world leader in clinical whole genome sequencing. The centre, which is a collaboration between the four universities Karolinska Institutet, KTH, Stockholm University and Uppsala University, was early in establishing its technical expertise, which is constantly evolving.
“Today we can sequence about 95 per cent of the genome and identify up to 60 per cent of the rare diseases, but our research and development will make us better, faster and cheaper,” says Valtteri Wirta, Head of Clinical Genomics at SciLifeLab and researcher at the Department of Microbiology, Tumour and Cell Biology, Karolinska Institutet.
How to find disease genes
Step 1. Blood sample
The patient’s DNA molecules are divided into many small fragments, approximately 300 base pairs long.
Step 2. Sequencing
The DNA sequence at the end of all fragments is determined in a machine. Thanks to the large amount of fragments, the entire genome will eventually be mapped.
Step 3. Computer analysis
Using powerful computers, the sequences of the fragments are placed in order against a reference genome of human DNA.
Step 4. Disease hunting
Deviations found in the patient’s DNA sequence are matched through an advanced database search of known diseases.
Step 5. Diagnosis
Clinical geneticists and physicians interpret data to determine which mutation is causing the disease in the patient.
Text: Fredrik Hedlund, first published in the magazine Medicinsk Vetenskap nr 1/2019.
Illustration: Amanda Berglund